00-02: The energy engineers' view on biomass for energy...
Stems and stalks, branches and twigs, foliage and roots
Biomass is in itself a wide concept including several different types of materials with different properties when regarded as a fuel. To get a first understanding to the diversity, a little bit of amateur level thinking around plant physiology may prove useful. It should be observed, that the following text is not a scientific essay from a plant physiologist but are the reflections of an energy engineer viewing some fundamentals in plants.
First: The main parts of any plant are the root(-s), the stalk or the stem with its twigs and branches and the foliage, be it needles or leaves.
The photosynthesis and the carbon loop
Starting from the foliage, that is where solar energy is stored by aid of the photosynthesis. The primary product of the photosynthesis is glucose which, in turn, is the building block for cellulose. Now; Cellulose has rather low energy content, as has its close cousin, hemicellulose. These two together are often referred to as holocellulose as they are both similar types of tall macro molecules. Holocellulose is then an early main product of the photosynthesis and it is not very energy rich in itself.
The word photosynthesis has not a unique meaning, but there are three different kinds:
• In temperate and sub-polar areas, the vast majority of naturally occurring plants use the so-called C3-photosynthesis for carbon fixation. Among these are the typical trees in the temperate and boreal climate zones, birch (betula), ash (fraxinus), beech (fagus), pine (pinus) or spruce (picea) just to mention a few. The process is called C3 because major intermediates are molecules containing three carbon atoms.
• To cope with hot and/or arid climates, another type of photosynthesis has developed, namely the C4-photosynthesis where the first intermediates are instead molecules with four carbon atoms. The C4-process uses an additional enzyme which is highly efficient to capture carbon dioxide from the atmosphere also when the concentration in or near the cell is low. In dry and hot climates, the plants will minimize perspiration by closing their stomata and in that case, the CO2-concentration inside the cells in the foliage becomes low. Thus, plants subject to such conditions – like sugar cane (saccharum officinarum), maize (zea mays) and several other commercially very important species – have developed the C4-photosyntehsis for their survival.
• Succulent and water-storing plants that are adapted to extremely arid conditions – cacti (cactaceae), for example – have developed another, even more sophisticated, process to make efficient use of carbon dioxide without loosing too much water through cell respiration – but these plants are of no interest from an energy point of view. These are called CAM-plants.
Regardless of the details in the photosynthesis process, the net effect is that carbon dioxide and water combine to store solar energy in the form of sugar which is then successively further transformed into cellulose, hemicellulose or other compounds. To transform carbon dioxide and water into glucose and cellulose, the plant needs use a number of enzymes, proteins and organic amino acids, chlorophyll being one of the most well-known actors.
What enzymes do is that they provide an energy efficient way to break up and recombine molecules and, typically, this occurs by the aid of metal ions and organic metal complexes.
The eternal carbon loop
Since carbon dioxide is taken up by the plants as they grow and since exactly the same amount of carbon dioxide is released as we burn the plants for energy purposes, the net effect becomes that biomass used as a fuel will be carbon dioxide neutral with respect to the atmosphere. With fossil fuels, carbon that was bound and stored underground millions of years ago is released into the atmosphere, with biofuel the carbon cycle is closed only over a few years. The time aspect is the crucial thing: A fuel or an energy system is considered carbon-dioxide neutral from a climate pint of view if the carbon cycle is closed in a shorter time than a few decades, and that is the case with biomass.
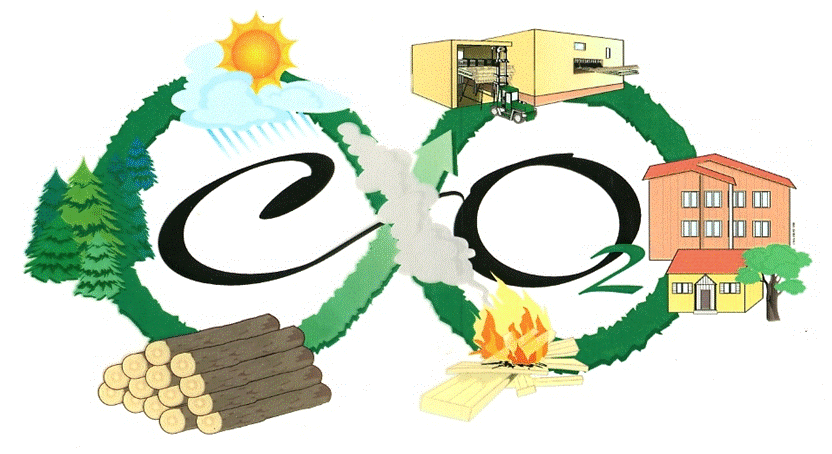
Different parts of the plant have different properties
In the foliage, where photosynthesis occurs, one will typically find lots of enzymes and, consequently, a comparatively high concentration of metals (which is ash to us energy engineers) and a rather high concentration of the primary products from the photosynthesis – which is holocellulose. Thus, the foliage typically will exhibit a comparatively low heating value in combination with comparatively high ash content.
The twigs, the branches and the stem are there to support the foliage and lift it so such a height where it can be effectively exposed to sunlight. At the same time, they shall serve as transport channels to supply the water and the metals and other nutrients needed for the photosynthesis. To do that, the material in the body of the plant must be porous and light while –at the same time – it must be rigid and flexible.
To achieve such a combination of properties, a honeycomb structure with composite walls would be preferred by most constructional engineers and this is also what plants have developed. The xylem – this is the name of the tissue specialized for transport of nutrients – in trees thus forms an outer zone of the stem. The central parts of the stem look slightly different. The xylem consists of tall cells with permeable membranes to provide for an efficient transport of water. For efficient transport, the ratio of internal cell volume to cell wall volume is low in this specialized transport tissue. As the stem grows thinner and we approach herbaceous plants, the xylem makes up a larger and larger fraction of the stem cross section.
So in a thick stem – like a tree – the porous and comparatively soft xylem is mainly found in the outer part of the stem, while the central parts are made up from a slightly more compact and rigid structure, the function of which is to provide the mechanical strength. In herbaceous plants, the stalk may be so thin as not to accomplish such a variation in structure over the cross section, and in that case the xylem will dominate the whole cross section.
Cellulose and hemicellulose are both chain molecules, cellulose built up basically by glucose units, maybe 5000 in a row, while hemicellulose exhibits shorter molecules – maybe 1000 – 2000 sugar molecule units – and hemicellulose also incorporates other sugars than glucose. Nevertheless, both molecules are tall and they lend themselves well to twist together to form fibres. As said just above, what the constructional engineer would want is to make up the cell walls by a composite material. Composites are made up by some type of a binder, reinforced by fibres. The main binder in botany is a polymer called lignin. So the cell walls are made up by lignin, reinforced by cellulose and hemicellulose fibres. Lignin – the glue substance – is a very complex and energy-rich molecule.
The approximate relations between the energy contents (usually referred to as lower heating values) between the three major components in biomass are: Lignin ΔH 25 MJ/kgDAF, Cellulose ΔH 16 MJ/kgDAF, Hemicellulose ΔH 15 MJ/kgDAF. In woody biomass – trees are big, and hence they need great mechanical strength and demand a lot of binder and glue – the order of magnitude is about equal shares of lignin, cellulose and hemicellulose and the heating value for stem wood becomes 18-19 MJ/kgDAF. Herbaceous plants are lower, lighter, do not need the same strength and hence they have less lignin and their stems and stalks do typically have a lower heating value.
In trees, the transport tissue for water, metals and nutrients mainly occupies an outer and minor zone of the stem cross section. Hence, the ash and mineral content in mature stem wood is typically low while the heating value is high due to the content of lignin. Bark, especially the inner bark which is an integrated part of the transport system in the tree – will typically have high ash content. As the transport route continues through the tree – from the thick stem to thinner branches, to twigs and to the foliage – will the proportions of xylem to mature wood and the proportions of bark to mature wood successively increase and, consequently, the heating value will tend to decrease and the ash content to increase. Of course there are exceptions to this: Coarse branches do often exhibit a high lignin content at their underside because the lignin is needed to provide support for the weight of the branch etc – but the above, simple reasoning will give the over-all picture and the general trends.
In herbaceous stalks the xylem may well be dominant across the whole cross section and then, obviously, the ash content becomes higher. At the same time will the heating value typically be lower with herbaceous stalks and stems than with tree stems.
The bark of a tree is there to protect the stem. Hence the bark needs be mechanically strong and the bark will typically contain a comparatively high proportion of lignin. At the same time is the innermost part of the bark also responsible for part of the water transport – and the ash content may thus be high. So bark will typically have a high heating value and relatively high ash content, relative to the stem wood, that is. For herbaceous plants, there is no bark.
The main role of the roots is to be the inlet for transport of water soluble nutrients into the plant. Hence what has been said about the xylem above is also applicable for the roots. For stumps of trees, though, age will also come in as an important factor. The lowest part of a tree is the part that contains the oldest wood and it is also the part that has been subject to the highest number of mechanical stress situations. As mechanical stress with a tree reaches critical levels, internal cracks may open and pitch streaks may form. To heal the wounds, the tree will typically produce extra lignin to reinforce the scar and such internal repairs will be found most frequently in the lower part of the stem. Hence, the stump of a tree may show a slightly higher heating value than the rest of the stem – due to a bit of extra lignin and resin – while the roots will typically show a lower heating value than the stem due to the high proportion of xylem.
Nuts and shells, berries and seeds, fruits and fibres
Utility plants – the plants dominating agricultural lands around the world – are grown mainly because of one, sometimes multiple, of the following five reasons:
1: The root, the berry or the fruit is wholesome, tasty and/or simple to prepare for food. In this group we may mention potatoes (solanum tuberosum) and carrots (daucus carota sativa) as examples of those where the root is major, strawberries (fragaria) or blackberries (rubus) as examples of berries and apples (malus) as well as pineapple (ananas comosus) as fruits. In botany, there is a strict difference between fruits and berries, but in this context we shall use the terms less strict.
2: The kernel of the nut or the seed is wholesome, tasty and/or simple to prepare for food. Examples of nuts are hazelnut (corylus avellana) and coconut (cocos nucifera) and for the seeds we may think of wheat (triticum) or rice (oryza sativa).
3: Sugar-rich juice can easily be produced from the root, the berry or the fruit. Sugar beet (beta vulgaris) and grapes (vitis) are two major examples.
4: Oil-rich or fatty juice can easily be produced from the kernel of the nut or the seed. Olives (olea europaea) and rape (brassica napus) are two examples.
5: Fibres can be easily produced from the stem, from the leaves or – in the specific case of cotton (gossypium) – from the flying aid of the seed. Again rape is a good example and hemp (cannabis sativa) is another.
Let's consider these qualities from the energy engineer's point of view.
For the first two cases, the plant reproduces via tubers or via pips, seeds, stones or kernels and the fruit or the berry or the nut are merely ways to provide for spreading of the genome.
In case the plant uses tubers for energy and nutrient storage – as is the case for potatoes, carrots or Jerusalem artichoke (helianthus tuberosus) and others – is the energetic use of above-ground biomass only a complement to the use of the main product from the cultivation.
In cases when the plant reproduces via any kind of seeds, the seed must contain sufficient energy in combination with sufficient nutrients and minerals to start a new plant even if it be situated below ground. Hence – typically – seeds of any kind, nut kernels, stones and others, will exhibit high heating values and relatively high ash content.
For the plant specie to spread there are several options, a very common one being to embed the seed in a tasty packaging – a fruit or a berry. The "strategy" (plants have no strategies since they have no consciousness – but biologists use the word strategy) is that the fruit will be eaten by birds or by land-living animals, the seeds will pass through the digestive system and hence the seeds will be spread across a vast area. To make the fruit or the berry attractive it must be easily digestible, it must be reasonably energy-rich or it may provide an enriched source of some specific trace element. Since the fruits and berries are thus easily digestible, they lend themselves well for fermentation to ethanol or for anaerobic digestion to biogas, and the residues will in both cases be rich in nutrients and lends themselves well as soil improvement agents or fertilizers.
The seed itself shall survive passing through the digestive system of the animal which means that the surface of the seed must be chemically resistive and this, in turn, implicates that seeds may be difficult to ignite if used in combustion applications. To improve ignition characteristics, crushing or grinding so as to expose interior areas are commonly used. As said previously, the seed is by definition energy rich but it is also rich in nutrients and minerals, so a high heating value and high ash content would be expected.
Packing the seed inside a rigid shell – nuts – represents another strategy. The "idea" in this case is that the nut can be physically transported across vast distances; coconuts may for example float across the Pacific Ocean and sprout as they are washed ashore. Hazelnuts may be collected by squirrels and other animals and hence transported to their winter habitat where they are cracked and those that are rejected and thrown out, or forgotten, may sprout in this new place. Thus, the nutshell must be rigid but not too brittle and it must be robust. Hence it is typically made up of the densest possible layer of fibres, glued together by as little binder as possible. Fibres, in botany, are holocellulose and the binder is lignin. Nutshells are only a packaging, so they need no extra storage of nutrients for future growth. Thus: Nutshells are composed of a large fraction of holocellulose, a little binder and only a little nutrients – hence the have low heating values and typically low ash contents.
To summarize
You will now understand that the most fundamental properties of biomass – regarded as a fuel – its heating value, its physical structure, suitability for biochemical treatment and its ash content are logically connected to the function of the specific biomass in the plant. Hence, biomass can be roughly classified in only a few groups go give a rough overview of the main property, which is the effective heating value or the energy content, expressed in MJ/kg dry, ash-free substance (ΔHDAF). The assortments mentioned in the table are only examples from the different groups, but they serve to give an indication of what types of materials in each major group (i.e. column) will have higher (top row) or lower (bottom row) heating values.
Agro-by-products | Woody biomass | Agro-industrial | Agro-biomass |
Rape seed refuse ΔH [27.5 - 28] |
Bark ΔH [19 - 21.5] |
Extracted presscakes ΔH [20.5 - 21.5] |
Canary grass (Phalaris) ΔH [18.5 - 19.5] |
Fruit kernels ΔH [17.5 - 23] |
Stem wood (conifer) ΔH [19 - 20.5] |
Press cake (olive) ΔH [17.5 - 19] |
Straw ΔH [17.5 - 19.5] |
Corn refuse ΔH [17 - 18] |
Stem wood (deciduous) ΔH [18.5 - 19.5] |
Press cake (grape) ΔH [16.5 - 17.5] |
Hay or miscanthus ΔH [18 - 19] |
Nut shells/rice husk ΔH [14.5 - 19] |
Poplar or salix (SRC1) ΔH [18.5 - 19] |
Cotton spills ΔH [16.5 - 17.5] |
Cotton stalks ΔH [15.5 - 18] |
Also, the order of the columns in the table is to roughly have the highest heating values to the left and the lowest to the right. The table is only indicative and aims to give a rough overview of some biomass assortments.
The next important property – according to the previous text – is the ash content. So why are there no indicative values for ash content in the table?
The ash content in a fuel as received consists of three major parts:
• Essential and major nutrients for the plant
• Trace elements necessary for the plant but present only in low concentrations
• "Logistic ash", contaminants that have accidentally been added to the material during handling, shipping, reloading and storing
What was briefly discussed in the previous text was the ash constituents denoted by the first two bullets but it is not at all uncommon that the logistic ash is dominant once the fuel reaches the energy plant.
Hence there is no general truth about total ash but the fuel supply system must be taken into consideration in each single case.
Quality aspects of biomass for fuel are, thus, complex and to bring some order into it it is important that anyone involved in the subject adopt a common terminology. Such a terminology is defined in the European federal standard EN 14588:2010 and a number of new standards are currently being produced for quality assurance throughout the whole bioenergy supply chain. Further references to standards can be found in the Forest StandardGuide.
You might also want to refer to the reports and guidelines published within the REGEN-project: The woodfuel standards guide, the manuals for processing, storage and logistics or maybe the guide for planners or the folder about contracting.
TEST what you have learnt!
INTRODUCTORY CHAPTERS 00-00: Global resources 00-01: Energy fundamentals 00-02: Download pdf 00-03: Fuel/Energy supply | ||||
FUEL↓ APPLICATION→ |
SINGLE-FAMILY HOUSES | LARGER BUILDINGS | DISTRICT HEATING | COMBINED HEAT-and-POWER |
FIREWOOD Introduction Properties |
Domestic firewood |
NOT APPLICABLE |
NOT APPLICABLE |
NOT APPLICABLE |
PELLETS and BRIQUETTES Introduction Pellet properties Briquette properties |
Domestic pellets | Large bldng. pellets | DH pellets |
NOT APPLICABLE |
WOOD CHIPS Introduction Properties |
NOT APPLICABLE |
Large bldng. chips | Chips for DH | Chips for CHP |