02-02: Herbaceous biomass – Resources
As explained in the Introduction to Chapter 01-02, herbaceous biomass is expected to be an important biomass resource for the future. In addition, its utilization may have a positive impact on a regional level, not only by displacing fossil fuels, but also by providing new jobs and income at a local level.
Mobilizing herbaceous biomass for bioenergy applications requires identification of potential resources. Generally, herbaceous biomass resources belong to one of two main
groups: agricultural residues and energy crops. For each group, the identification of resources raises different issues:
• Agricultural residues are on-field by-product of the production of food, fibre or feed crops. Some are collected for various end-uses, mostly animal feeding and
bedding, but others are not utilized at all. In any case, even though statistical data exist for the main agricultural product, residues are not thoroughly documents and it
is not always clearly understood what is available in a region and how much can be used for bioenergy applications.
• Energy crops on the other hand are grown as a main product by farmers and are intended for use in the bioenergy sector. Since energy crops are a rather recent
development, the issue here is mainly the applicability of energy crops for a given area and the factors that influence the decision for their introduction in the
agricultural sector.
A third group is the agro-industrial residues: by-products of the processing of crops that are produced by food industries. Although not always herbaceous in nature, they
are examined in brief in section 02-02-03.
02-02-01: Resources – Agricultural residues
The term "agricultural residues" refers to the parts of the crop that are not harvested during standard agricultural operations and are usually left on the field. The most typical and well-known example would be straw from cereals (wheat, barley, rye, oat), but also maize residues, rice straw, cotton stalks and others.
Typically, such residues have no nutritional value for humans and their application in processes that produce commodities (such as paper production) may be possible but it is fairly limited. This would give the impression that such residues are readily available for bioenergy applications. However, many of the most important residues, such as straw, do find application as materials for animal feeding and bedding, mushroom cultivation. Also, their removal from the field may be subject to environmental constraints, such as soil reservation.
As indicated in section 02-00-03 there are two main ways to approach the issue of
estimating the available for bioenergy applications quantities of agricultural residues:
• The first option is to collect data for the production of primary products of the agricultural activities in a region and then estimate
the production of residues by using certain ratios and assumptions for their availability. The steps required for the application of this method
and some key considerations are presented in the following paragraphs.
• The second option is to collect primary data on the production of the residues themselves, mostly by interviewing the actors involved
with the materials: farmers, contractors for their collection, logistic companies, end-users, etc. This method has the advantage that the info
collected is much more relevant to the conditions prevailing in a given region and reflect actual market practices. However, the quality of
some data may not be guaranteed, the method itself is more time consuming and costly and, most importantly, it may fail to produce any results
for materials that do not have any other end-use but are disposed, e.g. by burning in the field.
It should be noted that agricultural activities can also be a source of biomass in the form of prunings or uprooting residues from tree plantations and orchards. This can be a very important source of biomass, especially for South Europe. However, the biomass fuel that comes from these operations cannot be characterized as herbaceous and its properties are essentially that of woody biomass (although its ash content may be higher than cleaner forest biomass fractions). For more information on prunings and uprooting residues, see sections 02-01-04 (on resources) and 03-01-02 (on supply chains, mostly harvesting).
02-02-01a: Agricultural residues: Residue to Product Ratio (RPR)
As indicated before, the exact quantity of agricultural residues produced in each region is not monitored in most cases and such (accurate) data are not available from any authority. The data that is monitored and available though refers to the land cultivated and the production (or the yield) of the primary products: wheat grains, corn grains, rice, cotton, etc.
For the states in the European federation, agricultural statistics are collected at different levels and published at the Commission home-page: http://ec.europa.eu/agriculture/statistics_en.
On a regional level, statistics may be found by the national statistics agencies, agricultural ministries or other sources. On an area level, such info may be available via local offices of regional agricultural departments, farmer associations and other similar sources.
The conversion from primary agricultural products to residues is usually performed using the Residue to Product Ratio, or RPR (also referred to as the Straw to Grain Ratio or other similar terminologies).
RPR is obviously crop specific (since each plant has a different physiology), but also depends on several other parameters, such as climate and weather conditions, soil quality and agricultural practices (e.g. the application of fertilizers). It is not always possible to produce accurate guidelines for the effect of different parameters on the RPR, since literature data provide a score of data, some of which are not always consistent, or even conflicting. Part of the variations may be due to the changing moisture content of the residue in different locations, which are not always reported in the source.
In any case, there are some typical RPR values that are often repeated in the literature and can be used with relatively safety. These are presented in Table 02-02-02, along with other characteristics of the crop residues.
It is also possible to directly measure the RPR ratio in a given area, for example by sampling plants in a given plot, separating by hand the grain and the residue and measuring the weight and moisture of each separately. Obviously, this method is more time consuming and more costly. Also, in order to get meaningful results, the process should be repeated for a number of years in order to compensate for uncontrolled variable parameters, such as weather conditions.
02-02-01b: Agricultural residues: Removal rate
The use of the RPR ratio gives, with a varying degree of certainty, the total production of residues in a given area. However, this does not mean that all this quantity can be removed of the field, since several constraints may apply. Such restrictions include the following: equipment limitations, plant variety and harvest height and environmental constraints.
Depending on the cutting height, a part of the standing plant may remain in the ground and become unavailable for harvesting. The pattern of the vehicles movement in the field during harvesting operations is also an important factor: if the farmers do not consider the value of the residues, then tractors, trucks and other machinery may run over them while on the ground and thus make the unavailable.
The environmental constraints relate mostly to the maintenance of the soil organic carbon and the prevention of erosion by incorporating crop residues back in the soil by tillage.
Different sustainable removal rates are reported in the literature. For maize residues for example, values as low as 35% or as high as 75% have been reported, depending on the tilling and harvesting method employed. If carbon is returned to the field via other methods, e.g. by applying manure, then higher removal rates may be possible. The optimal removal rate for a given area would have to be determined by field data. For a first estimation though, the values given at Table 02-02-02 can be used, which basically amount to 40% removal rate for cereals and 50% removal rate for maize, rice, rapeseed and sunflower.
Crop | Residue Type | Harvesting Period | RPR | Removal Rate (%) | Residue Moisture | HHV, db | LHV, ar |
(% wt) | (MJ/kg) | (MJ/kg) | |||||
Soft Wheat | Straw | June | 1.30 | 40 | 15 | 17.90 | 13.74 |
Durum Wheat | Straw | June | 1.30 | 40 | 15 | 17.90 | 13.74 |
Oat | Straw | June | 1.30 | 40 | 15 | 17.90 | 13.74 |
Barley | Straw | June | 1.30 | 40 | 15 | 17.90 | 13.74 |
Rye | Straw | June | 1.30 | 40 | 15 | 17.90 | 13.74 |
Rice | Straw | October - November | 1.40 | 50 | 25 | 16.70 | 11.16 |
Maize | Stalks, Cobs, Leaves | August - September | 1.00 | 50 | 25 | 17.79 | 11.73 |
Sugarbeat | Leaves, Collar | August | 0.40 | 80 | 80 | 14.60 | 0.71 |
Tobacco | Stems, Leaves | October | 1.00 | 85 | 85 | 16.10 | 0.14 |
Cotton | Stalks | October | 2.00 | 50 | 45 | 18.20 | 8.31 |
Sunflower | Stems & Leaves | September | 2.00 | 50 | 40 | 20.80 | 10.64 |
Rapeseed | Stalks | September | 1.7 | 50 | 40 | 17.6 | 8.8 |
Table 02-02 1: Characteristics of main agricultural residues in the EU, along with typical properties |
02-02-01c: Agricultural residues: Competing uses
After finding the quantity of agricultural residues that can be removed from the ground without affecting the sustainability of the agricultural operation, the alternative to energy production uses of residues need to be identifying.
Many types of agricultural residues do not really have a competing use to bioenergy. Their fate depends on the crop type and the adopted agricultural practices; some are mulched and ploughed back in the soil, although far more common is that the farmers gather them and burn them in open-field fires. In some cases, such as cotton stalks, this is done to avoid pests and diseases to the plants that can be caused from the decomposing residues. However, open-field burning is a completely uncontrolled combustion, with the potential to form dioxins and other pollutants, and without any sort of energetic utilization of the produced heat. Therefore, if the logistics can ensure that these residues can be delivered to an energy plant at an acceptable cost, it is good practice to do so. If the costs are prohibitive, then the local authorities might wish to consider some form of financial support.
On the other hand, some other types of residues do have alternative uses and that means that bioenergy production has to compete with them. Straws are the most important of these residues and their most important uses are animal feed and bedding. Mushroom cultivation is another potential use; straw is used as a substrate along with manure or chicken litter.
Some residues can also be used as feedstock for various other purposes (e.g. pulp and paper production or houses built from straw bales), but such applications are not widespread and have no effect on the availability on a country level – on a regional or local level, if there are such competing uses, they should be dealt on a case by case basis.
The use of straw for animal feeding and bedding depends on several factors, such as straw availability itself (if it is too low, alternatives are sought), the number of livestock, the size of farms, the duration of time that the animals stay in the barns, etc. Barley and oat straw have a higher nutritional value and are preferred. Maize residues can also be used, but due to their low nutritional value they are not a priority; therefore, unless there is shortage of straw from other sources, the whole recoverable quantity can be used for bioenergy projects.
In order to estimate the local consumption in an area, the following figures can be used, which are taken from literature data and for
European conditions. Straw consumption tends to be higher in the cold North than in the South, so it would be better to adapt these figures to
local conditions, e.g. by interviewing local farmers.
• 1.5 kg of straw/day per head of cattle, used by 25% of the cattle population
• 1.5 kg of straw/day per horse/mule
• 0.1 kg straw/day per head of sheep
• 0.5 kg of straw/day/head of pig, used by 1/8 of the pig population
02-02-01d: Agricultural residues: Supply chain and yearly variations considerations
There are two more factors that limits the available resources of agricultural residues that can be used in bioenergy applications: the effect of the supply chain and yearly variations
Some comments on factors that limit the availability during harvesting / collection were mentioned in 02-02-01b, however there are other steps along the supply chain during which a part of the material is lost. The most important of those is the storage step, with transport and handling coming second.
The actual amount of losses during the supply chain depends on several parameters: plant type, moisture content upon harvesting, weather conditions, systems used, etc. Chapter 03-02 examines in detail the different steps of the supply chain and discusses possible sources of material loss. Typical values of dry matter losses for different storage options are presented in Table 03-02 2; as can be seen, they may be as low as zero or as high as 35%. Handling losses again depend on the actual conditions, but for a first estimation a conservative approach would be to use a loss of 5%.
Annual variations are another factor that has to be considered when estimating the availability of agricultural residues. Variations are mostly due to climate conditions which affect the biomass growth rate (this will also be reflected in the main product yield) and to the land area used for the cultivation of the main product (this information will also be available from existing data). Other parameters, include changes in the consumption patterns of alternative markets (e.g. the amount of straw used for animal feeding) or the effect of policy decisions (e.g. how much straw has to be ploughed back in the soil).
Figure 02-02 1 presents the variations of the total straw production and its various end uses in Denmark. As can be seen, not only the total production but also the amount use for various purposes varies over the years.
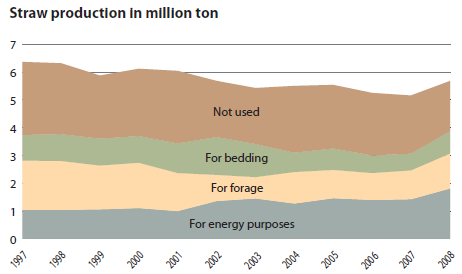
Figure 02-02 1: : Annual production and uses of straw in Denmark for a period of years (Source: Straw to energy, 2011)
It should be noted that the heating value of a biomass fuel is not constant but is also subject to the effects of the supply chain (mostly on its impact on moisture and ash content but also due to degradation of the material that directly affect the heating value) and annual variations (e.g. weather conditions that affect the moisture content, changes in growth pattern that affect the biomass composition and its heating value). See section 02-02-04 to see how a system planner should take into account all annual variations when dimensioning an herbaceous biomass energy system.
02-02-01e: Agricultural residues: Example of availability estimation and potential in the EU
This section presents a simplified calculation for the availability in the EU of two types of agricultural residues: cereal straw and maize residues. The calculation follows the methodology described in the previous sections and can be used as an example for a system planner who wishes to perform a similar assessment on a regional / local level.
Statistical data are taken from the Agricultural and Rural Development Statistics of the European Commission and are presented in Table 02-02 2 and 02-02 3.
Atlantic | Boreal | Continental | Mediterranean | ||||
Belgium | 273 | Estonia | 309 | Austria | 575 | Bulgaria | 1.375 |
Denmark | 1.443 | Finland | 1.201 | Czech | 1.375 | Cyprus | 31 |
France | 7.227 | Latvia | 518 | Germany | 6.043 | Greece | 903 |
Iceland | Lithuania | 939 | Hungary | 1.556 | Italy | 2.242 | |
Ireland | 289 | Norway | Poland | 6.764 | Malta | 0 | |
Luxenburg | 26 | Sweden | 979 | Romania | 2.928 | Spain | 5.496 |
Netherlands | 200 | Slovakia | 2.928 | ||||
Portugal | 171 | Slovenia | 58 | ||||
UK | 3.004 | Switzerland | Table 02-02 2: Land cultivated with cereals (common wheat + durum wheat + barley + oat), in 1000 ha (2009 data) |
Atlantic | Boreal | Continental | Mediterranean | ||||
Belgium | 9.04 | Estonia | 2.77 | Austria | 4.66 | Bulgaria | 3.50 |
Denmark | 6.85 | Finland | 3.55 | Czech | 4.88 | Cyprus | 1.84 |
France | 7.21 | Latvia | 3.14 | Germany | 7.07 | Greece | 2.50 |
Iceland | Lithuania | 3.56 | Hungary | 3.61 | Italy | 3.44 | |
Ireland | 5.93 | Norway | Poland | 3.37 | Malta | 0.00 | |
Luxenburg | 6.15 | Sweden | 5.10 | Romania | 2.30 | Spain | 2.42 |
Netherlands | 8.67 | Slovakia | 3.77 | ||||
Portugal | 1.58 | Slovenia | 3.69 | ||||
UK | 7.31 | Switzerland | Table 02-02 3: Grain yield of (common wheat + durum wheat + barley + oat), in tn/ha (2009 data) |
The harvestable yield of straw is calculated from Table 02-02 3 by multiplying with the appropriate RPR ratio of 1.3 and assuming a 40% removal rate. Then, the yield is multiplied by the land area in order to give the quantities of straw that are available for various market uses. Results are presented in Tables 02-02 4 and 02-02 5.
Atlantic | Boreal | Continental | Mediterranean | ||||
Belgium | 4.70 | Estonia | 1.44 | Austria | 2.43 | Bulgaria | 1.82 |
Denmark | 3.56 | Finland | 1.84 | Czech | 2.54 | Cyprus | 0.96 |
France | 3.75 | Latvia | 1.63 | Germany | 3.67 | Greece | 1.30 |
Iceland | Lithuania | 1.85 | Hungary | 1.88 | Italy | 1.79 | |
Ireland | 3.08 | Norway | Poland | 1.75 | Malta | - | |
Luxenburg | 3.20 | Sweden | 2.65 | Romania | 1.19 | Spain | 1.26 |
Netherlands | 4.51 | Slovakia | 1.96 | ||||
Portugal | 0.82 | Slovenia | 1.92 | ||||
UK | 3.80 | Switzerland | Table 02-02 4: Harvestable cereal straw yield in tn (wet) /ha (based on 2009 data and calculated using parameters from Table 02-02 1) |
Atlantic | Boreal | Continental | Mediterranean | ||||
Belgium | 1,284 | Estonia | 446 | Austria | 1,395 | Bulgaria | 2,504 |
Denmark | 5,141 | Finland | 2,215 | Czech | 3,487 | Cyprus | 30 |
France | 27,103 | Latvia | 844 | Germany | 22,207 | Greece | 1,174 |
Iceland | Lithuania | 1,738 | Hungary | 2,921 | Italy | 4,013 | |
Ireland | 891 | Norway | 0 | Poland | 11,849 | Malta | 0 |
Luxenburg | 83 | Sweden | 2,597 | Romania | 3,494 | Spain | 6,908 |
Netherlands | 901 | Slovakia | 1,199 | ||||
Portugal | 141 | Slovenia | 111 | ||||
UK | 11,425 | Switzerland | Table 02-02 5: Cereal straw available for market uses, in 1000 (wet) tn (based on 2009 data |
As can be seen from the tables, straw yields are higher in the Atlantic climate zone, followed by the Continental zone and lower for the Boreal and the Mediterranean ones. Taking into account a straw moisture of 15%, the averagy dry yield for EU-27 is 3.85 tn/ha.
For the available resources, not only yield but the cultivated land is also important. France and Germany, followed by Poland and the UK produce the most straw. However, in order to estimate the resources available for bioenergy applications, the current uses of straw in animal feeding and bedding have to be considered. In this calculation, only the straw consumed by cattle is taken into account: 1.5 kg of straw/day per head of cattle, used by 25% of the cattle population (see section 02-02-01c). The number of cattle per country is taken from Table 02-03 5. After some calculations, the straw available for bioenergy in each country is presented in Table 02-02 6.
Atlantic | Boreal | Continental | Mediterranean | ||||
Belgium | 928 | Estonia | 413 | Austria | 1,121 | Bulgaria | 2,426 |
Denmark | 4,930 | Finland | 2,090 | Czech | 3,302 | Cyprus | 22 |
France | 24,476 | Latvia | 792 | Germany | 20,435 | Greece | 1,089 |
Iceland | Lithuania | 1,632 | Hungary | 2,825 | Italy | 3,130 | |
Ireland | < 0 | Norway | 0 | Poland | 11,069 | Malta | - |
Luxenburg | 56 | Sweden | 2,386 | Romania | 3,127 | Spain | 6,084 |
Netherlands | 354 | Slovakia | 1,133 | ||||
Portugal | < 0 | Slovenia | 47 | ||||
UK | 10,070 | Switzerland | Table 02-02 6: Cereal straw available for bioenergy, in 1000 (wet) tn (based on 2009 data) |
The reduction is usually in the range of 7 – 15%, although there are cases where the methodology estimates even higher reduction (e.g. 28% for Belgium or 58% for Slovenia) or even negative availability, as is the case with Ireland and Portugal. However, it should be stressed out that these values are only indicative and valid under specific assumptions for the RPR, the removal rate and the cattle consumption of straw. Also, other markets and end-uses of straw were not considered, as well as yearly variations.
The results do not present a clear picture on a regional level. For example, straw may be produced in areas where there are not so many cattle farms, and hence it will be more easily available. Also, cattle may be fed with other types of plants or the consumption patterns may be different.
It should be noted that availability on a country level is not the only factor influencing the decision on the utilization of a given straw residue. For example, considering that the above calculations are valid, the available straw is actually less in Denmark that it is in Spain. However, Denmark is well known for its utilization of straw – mostly as a result of the policy direction - while straw-to-energy plants in Spain are a relatively recent development.
A more comprehensive estimation, taking into account several more factors as well as a regional analysis, of the potential of agricultural residues in Europe was performed by the Biomass Futures project. The results of the estimation for agricultural residues from cereals, maize, rice, sunflower and rapeseed are presented in Figure 02-02 2. The project foresee a doubling of the potential over the 2004 values (from 23 Mtoe to 49 Mtoe) as a result of increased production of cereals and a decline in livestock numbers. The agricultural residue potential is well spread over practically all of the EU, but on a country level it is concentrated in France, Germany, Poland, Italy, Hungary and the UK.
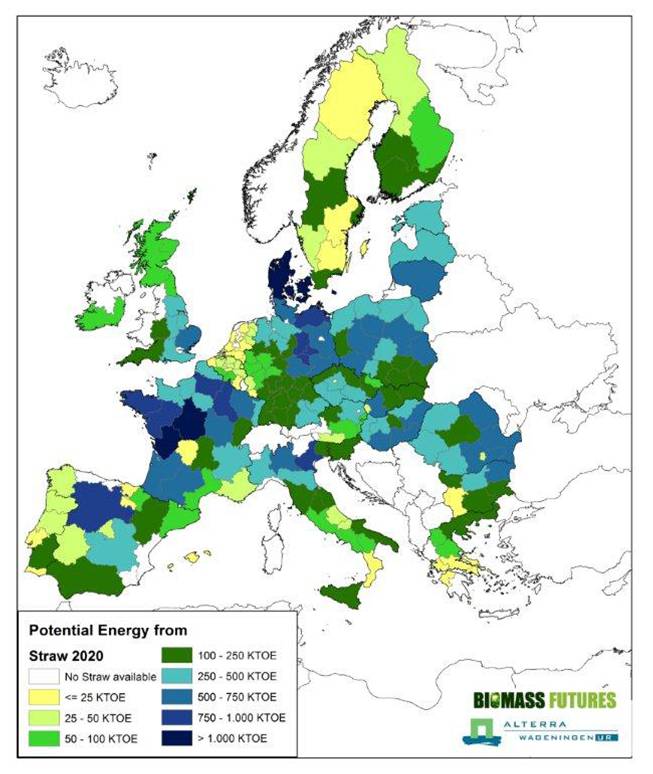
Figure 02-02 2: EU-27 economic and environmentally sustainable straw potentials (ktoe) in 2020 (Source: Biomass Futures project)
02-02-02: Resources: Energy crops
Energy crops are a relatively new development in agriculture. The term refers to any crop that is cultivated primary for its utilization as a feedstock for the production of an energy carrier, such as liquid biofuels, biogas or direct combustion for production of heat and power.
There are several reasons why energy crops have grown in popularity in the recent years. The most important is the potential for a high yield of biomass per ha with a careful selection of a crop type to each situation. This means a higher net energy production per ha and, therefore, better overall economics. From the various crop types, a selection can be made taking under consideration not only the local growing conditions but also the requirements for the process properties. In addition, agricultural inputs are lower compared to traditional crops and thus they allow for better utilization of fertilizers and water resources. Finally, some energy crops can be cultivated on marginal soils and thus bring them back to the productive economy, while increasing carbon storage in the soil.
Contrary to agricultural residues, the availability of energy crops depends mostly of the achievable yields in a given location. Although part of the material may not be harvestable (e.g. due to cutting height or for soil conservation) and supply chain losses due occur, there are no competitive uses and almost all of the crop yield can be used for bioenergy production.
The following sections examine some key points for energy crops.
02-02-02a: Energy crops: Types
Energy crops can be classified in different categories, depending on growth and cultivation patterns, plant types and intended use.
Growth and cultivation patterns significantly affect the biomass logistics and the type of agricultural activities that have to be performed each year. The following main categories can be discerned:
• Perennial energy crops,which are herbaceous plant species for which the life-cycle of a plantation extends over a number of years, usually 10-15. Such species
include many well known energy crops, like miscanthus, switchgrass and giant reed, as well as other crops lesser known crops, such as cardoon.
Perennial crops have the advantage that they require no annual tillage or planting; care of a year in the form of irrigation, fertilization, disease/pest control may be
required, but the initial costs for establishing the plantation are spread throughout the whole lifetime of the plantation. On the other hand, the long lifetime of the
plantation may seem to some farmers as a long-term commitment with high uncertainties – especially in cases when there is not yet a clear picture of the end product market.
• Annual energy crops grow and die within a year or a season and have to be planted again each time. Examples include fiber sorghum, kenaf and hemp, as well as most
of the traditional crops used for energy purposes (e.g. cereals, maize, sunflower, rapeseed).
• Short Rotation Coppice (SRC) are fast growing tree species, grown on arable land and under an intensive management including fertilizing, irrigation and weed/pest/disease control.
Typical examples include poplar, willow and eucalyptus, while there are also some lesser known types, such as paulownia.
In terms of land use, SRC may occupy lands previously used for agricultural products; their cultivation is therefore something that is undertaken by farmers and their introduction to an area relates mostly to the agricultural sector. However, in terms of the properties of the produced material, SRC is essentially a woody biomass, which is considered in more details in row 1 of this handbook. For further information on SRC, the reader is referred to section 03-01-03.
The energy crops considered as most appropriate for European climatic conditions are either C3 or C4 plants (see section 01-00-02a for more information on these photosynthetic paths). Generally, C3 plants are better adapted to grow under low temperature, and thus are usually cultivated in Northern European countries, while C4 plants are more efficient in high light and temperature levels, and thus are better suited for Southern Europe. Examples of C3 plants are giant reed, rapeseed, hemp and cereals, while maize, fiber sorghum, miscanthus, switchgrass and cardoon are C4 plants. Most SRC considered for Europe are C3 trees: willow, poplar, eucalyptus, however, some research is ongoing with C4 plants as well, such as paulownia.
A final categorization of energy crops relates to their intended end-use. The following categories may be distinguished:
• Oil crops, which are cultivated for their oil-rich seeds and which are used for the production of biodiesel. The majority of the energy crops currently
cultivated in Europe are oil crops, such as rapeseed and sunflower, due to the policy measures of that support the production of liquid biofuels.
The production of biodiesel from oil crops is a relatively easy process. However, since the oil that can be produced by such crops is edible by humans and the cultivation of such crops is done on arable agricultural land, serious concerns over the sustainability and the food vs fuel controversy have been raised.
• Sugar and starch crops,, which are used as feedstock for the production of bioethanol or biodiesel. In some cases, they are also used as raw material in biogas power plants (see 04-02-07). Examples include sugar beets, sorghum and maize and cereals grown for energy purposes. Their cultivation raises similar issues with the case of oil crops.
• Ligno-cellulosic crops,which are rich in carbohydrate polymers (cellulose and hemicellulose) and phenolic polymers (lignin). Such crops can be used as solid biofuels in thermal processes (see Chapter 04-00 for a general introduction or Chapter 04-02 for more specifics on processes for herbaceous biomass). They can even be considered as 2nd generation feedstock for biofuel production, although in this case the process is more complicated than oil and sugar crops (see 04-02-06).
Examples of lingo-cellulosic crops are miscanthus, switchgrass, hemp, as well as the SRC species. Overall, lingo-cellulosic crops tend to raise less alarm over the food vs fuel issue, especially if they are grown on marginal lands.
It should be noted that the categorization is not always straightforward. For example, cardoon can be grown as an oil crop (e.g. for its seeds) or as a lingo-cellulosic crop (in which case the whole plant is used as feedstock in an energy process). Oil crops, such as rapeseed and sunflower, also leave residues that can be used as a lingo- cellulosic feedstock. Some harvesting methods that allow for the simultaneous collection and separation of different crop parts are currently being developed, although mostly they are still in the design phase.
02-02-02b: Energy crops: Selection and yields
Given the existing policy schemes, energy crops are considered an attractive feedstock for the production of bioenergy carriers for one of two main reasons: biomass composition and achievable yields.
The biomass composition is currently the most important factor for the production of liquid biofuels. Given that there are still technical restrictions in utilization 2nd generation biomass (e.g. lingo-cellulosic biomass) for the production of liquid biofuels, most conversion plants utilize 1st generation feedstocks that are rich in oils or sugar/starch.
In the long-term however, it is expected that technology advances and appropriate pre-treatment technologies will allow the production of liquid biofuels from ligno- cellulosic feedstocks, which can also be used for direct production of heat and power in thermal processes. Ligno-cellulosic energy crops can achieve much higher yields than oil crops. Even compared to the typical yields of most agricultural residues (for example, 2 – 4 tn of dry matter per hectare for straw), perennial energy crops and SRC have reported yields that range from 5 up to 30 tn of dry matter per hectare, although most fall somewhere between 8 – 15 tn of dry matter per hectare. These yields result in higher collection densities and improved logistics and overall fuel costs (see also Chapter 03-02).
The choice of energy crops for a given region depends on several parameters, the most important of which are:
• Climatic considerations (rainfall / droughts, temperature range, and resistance to droughts). Depending on those, crops may be chosen based on their resistance to
cold or droughts or their water uptake requirements.
• Soil conditions, e.g. whether the crop is capable of growing on marginal lands or whether it requires arable land.
Apart from those, other important parameters to consider include:
• Existing varieties suitable to the region (breeding activity).
• Means of propagation. Propagation by seed is easily mechanized and leads to lower costs.
• Knowledge of agronomic practices (soil tillage, sowing methods, fertilisation, crop protection, harvest time).
• Mechanization and suitability of existing agricultural equipment.
• Supply chain issues, mostly the existence of transport and storage infrastructure.
• Farmer’s acceptance. As noted in 02-02-02a, farmers may be more acceptant of annual crops than perennial crops.
• Biomass properties, such as ash content and heating value. Herbaceous energy crops have typically quite high ash values and chlorine concentrations, which may pose
significant issues in several conversion technologies such as combustion and gasification (see Chapter
03-02 and the Ash Appendix for more information). The difficulty in
finding suitable conversion processes has been an important limiting factor for the spread of such crops.
Overall, the target is to achieve high and stable yields and high energy production per unit of surface area with a minimum of input (irrigation, fertilizers, pest/disease control). Several publications provide indicative yields for different energy crops types (see the References section for examples). It should be kept clearly in mind however that reported yields (as should be obvious from the discussion above) are highly variable and depend on a number of conditions. Therefore, the transferability of results from other area to another should be carefully evaluated by experts before any decisions are made.
It should also be noted that the yields and quality characteristics of energy crops exhibit annual variations, just as in the case of agricultural residues. See section 02-02-01d for more information.
02-02-02c: Energy crops: Current and future status in the EU
As a result of several policy measures that support the production of biofuels for the transport sector, as well as bioenergy in general, the agricultural land on which energy crops are grown has risen in recent years to 5.5 million hectares. Most of this land is cultivated with oil crops for biodiesel production (82%) or crops that are used for the production of bioethanol (11%), mostly in France and Germany but also in the UK, Poland and Romania. Crops grown as feedstock for biogas production also take up an important part of that land (7%), especially in Germany, while perennials and SRC for electricity and heat generation are still only a minor part (1%).>
The EU target for 2020 is that biofuels should account for 10% of the energy used in the transport sector. According to calculations of the European Commission (2008), this target would require 17.5 million hectares of land, or 10% of the total Utilized Agricultural Area (UAA) in EU27. Considering the area required for feeding the population of EU27, it is estimated that 10-30% of the arable land could be used for the cultivation of energy crops. In addition, marginal lands that are not suitable for food production could also be used. The calculation assumed that 50% of the production would come from cultivation of rotational biomass crops for 1st generation technology biofuels., while the other 50% would come from 2nd generation feedstock (residues, perennial energy crops and imports), for which there will be available conversion technologies by 2020. Other estimations from the OECD are less optimistic and bring the required land area to 45 million hectares of land, however they assume only 1st generation biofuels and current yield levels.
An overview of the potential for energy crops in the EU, as estimated by the Biomass Futures project is presented in Figure 02-02-03. The regional distribution of dedicated cropping patterns is based on the assumption that the bioenergy crops are distributed over regions in the same proportion as similar crops are used for feed and food purposes.
The issue of sustainability has been raised for energy crops much more than for any other type of biomass (agricultural residues, woody biomass, waste, etc). This issue does not only relate to the environment but also to social issues, such as the food vs fuel controversy. More information on sustainability issues is presented in Chapter 02-01.
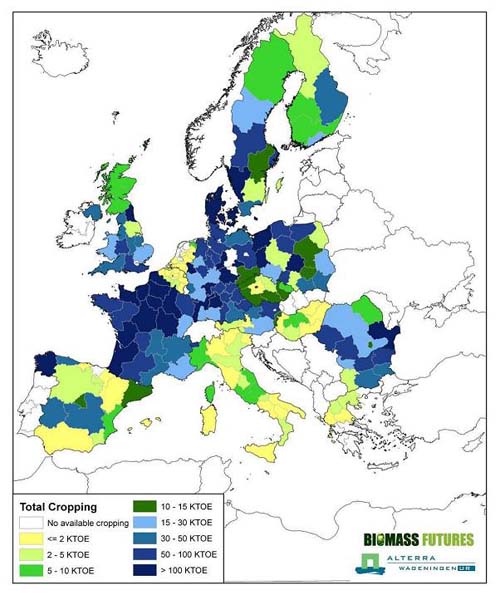
Figure 02-02 3: Cropping potentials for energy crops in Europe (Source: Biomass Futures project)
02-02-03:Resources: Agro-industrial residues
Agro-industrial residues are a final type of biomass resource that is connected with the activities of the agricultural sector. Unlike agricultural residues or energy crops, which are both available on the field and must be collected over a wide area, agro-industrial residues are by-products of an industrial process, usually related to the food or fiber industry. As a result, such residues are easy to collect from a specific point and their logistics are greatly simplified
Examples of agro-industrial residues include kernels from fruit canneries and olive oil production plants, cotton ginning residues, husks from rice, nuts, coffee, oily seeds and others. The organic residue of certain bioenergy process, such as the lignin fraction of biochemical fermentation (see for example 04-02-06), could also be considered as an agro-industrial residue.
Not all agro-industrial residues are actually herbaceous in nature; the lignin content of many of them such as olive kernels or husks is as high as or higher than wood fuels. Depending on the process from which they are produced, their moisture content can also be quite low. As a result, many of these residues are actually an excellent combustion fuel. However, there may be some problems due to a quite high chlorine content (see the Fuel Appendix and the Ash Appendix for the impact of chlorine in combustion processes). It is quite common for such residues to produce at least part of the heat required for the processing of the main agricultural product (e.g. cotton drying), while the remaining are often sold as a heating fuel for other industrial or domestic applications. This “process integration” of the agro-industrial residue is also an important part in bioenergy process: e.g. the non-convertible lignin fraction of a straw that is turned in a liquid biofuel is burned and the heat is used to cover the energy demands of the process itself (e.g. distillation or pre-treatment).
The availability of agro-industrial residues on a European level has not been as widely studied as that of agricultural residues or energy crops, perhaps due to the fact
that they in several cases already utilized as an energy resource. The actual amount of residues produced by a given process depends not only on the quality of the incoming
product but also on the process itself. Some reported values are:
• Olive husks are approximately 23% of the olive oils. The moisture is variable depending on the process and can be up to 30% - usually though it is much lower.
• Rice husks are approximately 16% of the rice, with a moisture content of 10%.
• Cotton ginning residues are approximately 10%, with a moisture content of 17%.
The yearly availability of agro-industrial residues varies depending on industrial process typical method of operation. Rice mills or peeling plants for dried nuts may operate throughout the year, and thus the residue is available in more or less steady quantities on a yearly basis. Other plants, such as olive mills operate only for certain months of the year; the residue is available at the time and it has to be stored if it is to be used any other time.
02-02-04:Planning aspects
This Chapter must have made clear that herbaceous biomass resources actually cover a wide range of materials, from different types of agricultural residues to dedicated energy crops. Basically, in every region whether agricultural production takes place, there will be some type of residue that can be used in bioenergy applications; even marginal lands can be incorporated into the agricultural sector by introducing suitable energy crops.
The first step in planning every bioenergy system is the inventory of currently available resources. In most cases, this means the consideration of available agricultural residues (or agro-industrial residues). As has been indicated in section 02-00-03, one strategy for doing so is to collect relevant data from surveys and interviews with local stakeholders. Such data are more relevant to the area in question but their collection is time consuming and many not provide such accurate data for by-products that are not currently used.
The other option is to use a methodology and typical literature values, such as the one described in section 02-02-01. Although straightforward and relatively easy to use, its results may not accurately reflect local conditions.
The best course of action would be somewhere in the middle: use the described methodology, but adapt values, such as the removal rate and the residue-to-product ratio, to local conditions, following consultation with stakeholders, expert opinion or results from demonstrative actions. The use of GIS tools can also be a useful approach for estimating agricultural residues at regional and local level.
Regardless of the type of resource - agricultural residue, energy crop, agro-industrial residue or any other type of biomass for that matter – it is not the biomass in itself that has a value but rather the potential to provide an energy service. In order to estimate this potential, one has to multiply the biomass quantity with the biomass heating value.
The means to identify the biomass quantity have been discussed in detail in the previous paragraphs. The heating value and the parameters that influence it is discussed in detail in 03-00-02c and 04-00-01a. What a system planner must realize however is that both quantities exhibit yearly variations due to the influence of a number of factors, such as weather conditions, supply chains variations, etc. Such variations tend to be much more pronounced for herbaceous biomass compared to woody or waste biomass.
Therefore, by considering a sufficient number of years (at least 10), it will be possible to calculate not only a mean annual yield and heating value, but also their respective standard deviations.
Dimensioning an energy plant for the mean annual yield will inevitably result in fuel shortages, since – by definition – there will be a feedstock deficit every second year. There will also be surpluses every second year as well, but since there are limitations in the long-term storage of biomass, it will not be possible to level out the differences by storing excess quantities.
The best approach is to dimension the system but considering the a biomass yield and heating value equal to the averagy value minus the standard deviation. Although this does not rule out completely the possibility of fuel shortages, it ensures that for the majority of the years there will be sufficient ”energy” delivered at the energy plant. It should be noted that yearly data for some of the required parameters may not be available if the bioenergy system is starting from scratch. It would not be possible for example to find historical local data on the ash content of straw, if no one was using it to produce energy. In this case, it makes sense to use literature values or expert estimations.
In the end though, the issue available herbaceous biomass resources in a given area returns to the more fundamental question of how to use a limited natural resource (agricultural land) to cover all of society’s needs. The agricultural sector is also a dynamic part of the world economics and its output changes depending on consumer needs and market conditions.
A basic principle for the long-term planning of herbaceous biomass resources is that a system planner should be mindful of competing uses – but also be aware of opportunities.
For many types of agricultural residues, such as straw, there already are competing, non-energetic, uses, mostly as animal feeding and bedding material. This means that bioenergy applications will have to compete with these applications for resources – and also to beat the market price they can meet there. Alternative resources could be considered for both possible utilization pathways – normally though, it is considered a good practice to avoid the competition with traditional markets and to utilize the resources than remain after all other demands are met.
However, many other types of residues are burned in the field and their use in bioenergy conversion process would be of environmental benefit. Replacing some low level biomass-to-energy systems with more advanced technological systems might also liberate some of the already utilized resources.
For energy crops, the issue is not that of their final use (since they are grown for energy production) but whether the agricultural land should be used for their cultivation in the first place. The answer is more complicated here, since it relates to sustainability issues (environmental and social), policy direction, the status of the agricultural sector and other factors. The introduction of energy crops in a given area is not a decision to be taken lightly.
However, energy crops can be an excellent solution for marginal lands, where it is not possible to cultivate any other crop.
There are two further points a system planner has to consider regarding the available herbaceous biomass resources at his/her disposal: the impact of herbaceous biomass fuel qualities (see Chapter 04-02) and also the sustainability and policy issues (see sections 01-02-03a, 01-02-03b, 01-02-03c and 01-02-04).
References
Further discussions on the methodology for the evaluation of the availability of agricultural residues presented
in this Chapter can be found in the following publications:
•
Nicolae Scarlat, Milan Martinov, Jean-François Dallemand, Assessment of
the availability of agricultural crop residues in the European, Waste Management, Volume 30, Issue 10,
October 2010, Pages 1889-1897
•
Esteban, L. S., Ciria, P., and Carrasco, J. E. (2008). "An assessment of relevant methodological elements and criteria for surveying sustainable griculcural and forestry
biomass byproducts for energy purposes," BioRes. 3(3), 910-928
For a more detailed examination of the methods used in evaluating biomass potentials, the following publication
is useful (which also reports values for residue to product ratios)
• Rosillo-Calle F., de Groot P., Hemstock S.L.,Woods J. (2007),
"The Biomass Assessment Handbook", Earthscan, London, United Kingdom.
Regarding energy crops, there are several reports available from EU-funded projects that present experiences,
yields and other considerations for different crop type and growing conditions. The present chapter was based on
information taken mostly from the following resources:
•
Energy from field energy crops - a handbook for energy producers,
published by the ENCROP project.
•
Various publications from the
RESTMAC project can be found
here.
•
The "4F Crops project" investigated yields and crop types but the original homepage is obsolete.
Over-all project information can be found here.
For a broader view of the role that biomass can play in meeting EU energy policy targets, as well as estimations on the future potential of herbaceous biomass, the reader is refered to the publications of the Biomass Futures Project. On a regional level, several European regions have produced Action Plans that emphasize the role of herbaceous biomass. See for example the Bioclus Project or, the Bio-En-Area subproject BIOREF on energy crops in particular.