03-00: Biomass for energy - Supply chains
One of the main differences between biomass and fossil materials is the simple fact that biomass remains a biologically living matter and it is also chemically active throughout the supply chain, while fossil fuels may be considered biologically inert. This will affect the risks during handling and storing and becomes manifest in the facts that biomass will deteriorate during storage because of biological activity while – at the same time – allergenic spores may accumulate in the fuel.
The biological activity will alter the composition of the material and will also have an influence on the moisture distribution in the piles of material. If stored in closed compartments the biological activity may deplete the air of oxygen causing an acute risk for suffocation in cases where the compartment is entered. Product gases from the deterioration may also be poisonous like carbon monoxide or hydrogen sulphide or explosive, like methane. The temperature rise caused by the biological activity may also, ultimately, trigger self-ignition.
The self-ignition process in biomass piles is not understood in detail but generally speaking it is believed that the initial phases are due to biological activity followed and partially acting in parallel with chemical, heat-releasing reactions.
With fossil fuels, there is also a risk for auto-ignition, especially in the case of coal storage, but in these cases the main cause is rather because of chemical reactions than of biological activity. Similarly, the emanation of hazardous gases from storage of fossil fuels is believed to be the result primarily of chemical reactions rather than of biological activity.
The second major difference between fossil fuels and biomass aimed for energy is the concentration at the point of origin, the location where it is exploited. For fossil fuels, the point of extraction is solely determined by the concentration and by the simple fact that the concentration is high enough to make production economically feasible. Hence, fossil fuels are, per definition, produced only at such locale where they are concentrated, be that at sea, in a desert or wherever.
The production of biomass for energy purposes is more complex since it may be – and today often is – part of a local or regional policy. So the production of biomass for energy may be part of a social policy to abate unemployment in a rural area, it may be part of a system to solve a major waste handling problem in an urban area, it may provide an extra income for a forest owner or it may be subsidized to stimulate a transformation of local or regional agriculture from one type of production into another.
Currently, the first decades of the 2000’s, when there is a rapid expansion of biomass use for energy purposes in countries and areas where the large-scale bioenergy production and use has no tradition, there are many examples worldwide when biomass aimed for energy production is produced in ways that are not economical in the long run. There are also examples where the urge for profitable biofuel production have led to the establishment of monocultures for energy production which may well put the biological diversity at risk and may hence not be ecologically sustainable. There are even examples when cultivation of energy crops has replaced cultivation of food and/or fibre crops.
To summarize, it is not necessarily so that the production of biomass for energy purposes is done under thorough consideration of the limitations outlined in chapter 02-00-02, but in some cases it may even be set in direct violation to the natural conditions and in these cases, usually at a significant cost.
Regardless, the availability of biomass will always and ultimately be limited by the biological production rate. As was seen in tables 02-00 4 & 5, for example, the growth rate of forest and crops is limited by climate, soil and a number of other factors, and this applies to all kinds of biomass – terrestrial and marine alike. So to “harvest” a specific amount of energy in the form of biomass, a large area needs be physically covered while to “harvest” a similar amount of fossil gas from a gas well you may just wait for a sufficient amount of gas without moving.
As consequences of this the cost distribution throughout the supply chain will become radically different when comparing biomass with fossil fuels and this will be described in some detail in chapter 5.
03-00-01: Terminology
Among those not professionally engaged with bioenergy systems, confusion with the fundamental terms is commonplace. Throughout this handbook, the terminology will strictly follow the supply chain as outlined in the European Federal standard EN 14588. The basis for this standard is outlined in the schematic below.
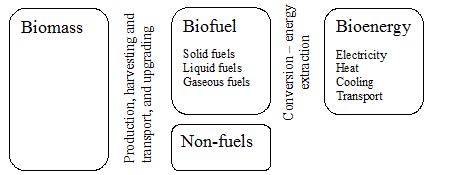
Figure 03-00 1: Terminology according to EN 14588
There are strict definitions for the terms in the standards documents but in short and for the purpose of this handbook the following will be sufficient:
Biomass is a substance with a biological origin that has not been chemically converted and has not been contaminated by any additives.
Biofuel is a combustible substance originating from biomass. The fuel may have undergone a chemical conversion such as gasification, liquefaction, torrefaction, fermentation, anaerobic digestion or alike or it may have undergone a physical conversion such as milling, chipping, pelletizing, briquetting or alike and it may likewise contain additives as long as these have not added heavy metals or other harmful substances.
Bioenergy is a (commercial) energy carrier originating from the use of biofuel. The energy carrier can take different forms such as bottle (bio-)methane, (bio-)ethanol offered at filling stations, electricity, heat in a district heating system, wood pellets or alike.
Strictly speaking, the energy finally distributed cannot be produced but was contained in the original biomass, stored by photosynthesis as outlined in 01-00-02. However, the word energy production is commonly used and for this handbook the following definition is used:
Energy production is (the inadequate) term used for the processes in which the energy contained as latent chemical energy in the (bio-)fuel is extracted and converted into a (commercial) energy carrier. The most common process by far is combustion, used with solid, gaseous and liquid fuels, but catalytically assisted; low-temperature oxidation in fuel cells can also be used.
Energy plants are those (industrial and commercial) plants where energy production takes place. Energy plants can be power plants – producing only electricity – heating plants, producing heat only, or combined heat-and-power plants (CHP-plants) producing heat and electricity simultaneously. The last kind of plants may also produce (district) cooling. Further specification and descriptions of the different processes will be given in chapter 4. For the scope of this chapter, the term energy plant is used in an inclusive way, including also plants for gasification, liquefaction or any other fuel transformation process.
The supply chain with biomass will have a significant influence on the properties of the biomass finally delivered. The difference between these properties is defined by the intrinsic and the extrinsic properties:
Intrinsic values: Intrinsic water content in a biomass is the equilibrium value at a certain temperature and relative humidity. Similarly the intrinsic ash content is the content of minerals and nutrients natural to the plant in question. So an intrinsic property can be considered as a reference value for the biomass in question when it is clean and in equilibrium with a surrounding.
Extrinsic values: Extrinsic water content in a biomass is what it holds when it has been subject to sudden, random, conditions such as a rainfall. Similarly the extrinsic ash content is that which is measured if the biomass has collected a lot of soil or road dust during the supply chain. So an extrinsic property can be considered as the representative value of the specific parameter for the biomass as delivered at the energy plant.
The difference between extrinsic ash content, moisture content and heating value and the corresponding intrinsic values can be seen as an over-all quality measure or the supply chain.
The scope of this chapter is to outline the sub-processes involved in the chain from raw, solid, biomass until it is delivered to a plant where it is either converted into a new type of fuel or the energy is finally extracted.
To clarify: Assume corn aimed for ethanol production as an example. Then this chapter will only discuss the supply chain of the corn and will not discuss the distribution of ethanol, since the latter is already a mature, well-established and fully commercial handling.
03-00-02: Unit operations
When harvested, biomass is solid with water and ash contents and particle sizes partly depending on the harvesting technique, partly on the season and partly on the biomass origin.
One of the most extreme ends may be represented by the harvest of algae and plankton for bio-methane production. The size of the algae and plankton may typically extend from less than a millimetre to some centimetres and the water content would typically well exceed 90 % by weight.
Another extreme may be represented of wheat straw, harvested (i.e. collected) some days after threshing, provided the weather has been dry. At certain conditions the moisture content may be about the typical intrinsic value, 15 %, and the ash content as low as about 4 %.
As will be clarified in chapter four, the energy extraction process – be it combustion, gasification, biochemical conversion or any other – will be designed to work properly only with biomasses within certain quality ranges. So there are always limits to ash content, ash quality, water content, heating value and particle sizes that have to be met for the system to work properly and the supply chain must be apt to meet these demands.
03-00-02a: Unit operations – Harvesting/Collection
Splitting the biomass into four major groups, forest and felling residues, residues from agriculture or aquaculture, residues from industrial processes and societal waste streams, one readily realizes that the former two fractions have many properties in common and so do the latter two.
For the first two groups, the solid biomass/fuel is a side-product to a utility that was already produced before, and the “harvesting” in such cases is a question of collecting something that was (in the case of straw, for example) or was not (in the case if thin branches at a felling site, for example) previously collected. From a logistic point of view this may then involve an additional operation on-site and it may even involve additional machinery.
In case of a combine harvester collecting and baling the straw simultaneous to threshing, the selling of the straw to an energy plant causes no extra cost as compared to any other use of it. There may be a hidden cost, though, because the farmer may have to replace the straw with some other, commercial, product for internal use at the farm. In this case, the additional logistics are fundamentally nil – while there still is an external cost that needs be considered since the side product originally had another use and the use for fuel is competing.
In aquaculture – as well as for some agricultural side-products – the competitive use may be one of great significance, like the use of algae or of press-cakes for fodder. This is mentioned only to make it clear that to understand the real cost for the harvested material, one has to understand and appreciate the different alternative uses.
Engaging a forwarder to collect the branches spread across a forest felling site, though, will be a costly arrangement and the full additional cost should be attributed to the fuel produced. In this case the logistics become very much more complex once fuel production is introduced compared to the original situation. At the same time, the side product (thin branches) actually had not market value until it was extracted for fuel and there are no hidden costs.
In some cases may the collection of industrial residues for energy be highly automatized, like using pneumatic transport from the individual dust-bins below the cyclones at a joinery to get all the saw-dust into one place. In other cases – such as the collection of putrescible fish rinsing from a food processing industry – the collection process may have to involve a change of the production logistics themselves.
The collection of clean societal and household waste fractions poses specific problems. For household waste, the quality, i.e. the cleanness, of the fuel fraction is completely determined by the separation obtained.
A good separation can be obtained in two ways: Either, responsibility is put onto the households to perform the separation or a designated sorting facility is established.
A combination used in some cities in Sweden is based on the households separating three different organic fractions (clean combustible, biodegradables and non-combustible) into different coloured bags. Materials outside these fractions – glass, metal, ceramics, paper etc – are handled in a separate way and will not be treated further here. The bags are all collected in the same compartment in the refuse collection vehicle. Separation of the bags is then done at a common, fully automatized sorting facility where also an occasional quality control takes place. The results from the quality control must be communicated back to the households to maintain a high degree of awareness that sorting is essential. At the same time, using only one truck with a common compartment for all fractions, keeps the investment and handling cost at a minimum.
For larger facilities – such as major lunch restaurants at university campuses or in larger shopping malls or for the collection of formwork timber or demolition wood from larger building sites – the quantities of specific fractions of waste may well be large enough to motivate a dedicated vehicle only for one fraction at a time.
Depending on the exact nature of the collection/harvesting process, there is always a risk of foreign materials entering the fuel fraction.
In many – most – cases when a fuel fraction is produced side-by-side with another fraction, such as straw for fuel produced aside corn production or forest felling residues being produced at the same time as timber, the fuel fraction will be placed on the ground while the harvesting of the main product commences. Doing this, it becomes very important that the operator is well aware that the fuel fraction is a product – not waste.
The drivers must be instructed not to drive across the fuel fraction but to put it clear of the driveways, otherwise the fuel fraction will be heavily contaminated by soil and its ash content will be significantly increased. Once a routine for fuel fraction handling has been established, it does not increase the handling time significantly – but the quality and the value of the fuel fraction is radically improved.
03-00-02b: Unit operations – Transport
The transport of biomass is typically limited by the energy (bulk) density.
Energy density is energy content per volume, not to be confused by the heating value which is the energy content per weight.
Provided a certain biomass quality is characterized by a certain (lower) heating value qNET (for explanation of the subscript NET you are referred to chapter 4) MJ/kg and a certain bulk density ρBULK you will obtain the bulk energy density qVOL by
qVOL = qNET * ρBULK MJ/m3.
The lower the energy density, the less becomes the total amount of biomass energy that can be loaded on board a carrier (a railway car, a truck, a boat…) with a limited loading capacity as expressed in volume. And the less the carrier capacity – the higher the transport cost.
From this expression it becomes clear that there are two ways to increase the load capacity of the carrier:
• Either can the lower heating value (qNET) be increased, for example by drying
or
• Can the bulk density (ρBULK) be increased, for example by pelletizing or by comminution. Both of these will increase the degree of packing and thereby the bulk density.
However, transport of biomass is not only a question of cost but may also have a significant influence on the quality. Transport on dry dirt roads, and this is often the case as harvesting of agricultural products as well as of forest residues often occurs in dry weather and in rural areas, may significantly increase the ash content of the fuel because of road dust settling on the fuel. The problem is easily eliminated by covering the fuel by a tarpaulin during transport.
03-00-02c: Unit operations – Drying
The heating value of biomass is determined by three factors independently,
• The composition of the dry, ash-free substance and the its heating value qNET,DAF
• The ash content, weight fraction fASH, dry basis
• The moisture (water) content, weight fraction fW, wet basis
For most biomasses, the dry ash-free substance consist of cellulose (qNET,DAF ≈ 16 MJ/kg), hemicellulose (qNET,DAF ≈ 16 MJ/kg), lignin (qNET,DAF ≈ 26 MJ/kg), extractives (qNET,DAF ≈ 30-35 MJ/kg) and fatty acids (qNET,DAF ≈ 30-35 MJ/kg) in variable proportions depending on the type of biomass. This will be dealt with in more detail in chapter 4. It is important to realize that the heating value of the dry ash-free substance cannot be accurately calculated by simply adding these values with the weight fractions as weighing factors but that such a simple addition will only give a rough estimate.
The table below – compiled from a number of sources – gives an indication of the expected heating values for a few different assortments of biomass:
Agro by-products | Woody biomasses | Agro-industrial | Agro biomasses | Rape seed refuse 27.5 - 28 |
Bark 19 - 21.5 |
Presscaces (extr) 20.5 - 21.5 |
Canary grass (phalaris) 18.5 - 19.5 |
Fruit kernels 17.5 - 23 |
stem wood (conifer) 19 - 20 |
Presscaces (olive) 17.5 - 19 |
Straw 17.5 - 19.5 |
Corn refuse 17 - 18 |
stem wood (deciduous) 18.5 - 19.5 |
Presscaces (grape) 16.5 - 17.5 |
Hay (miscanthus) 18 - 19 |
Nutshells/Rice husk 14.5 - 19 |
Poplar (salix, SRC1) 18.5 - 19 |
Cotton spills 16.5 - 17.5 |
Cotton stalks 15.5 - 18 |
Table 03-00 1: Indicative values of qNET,DAF, MJ/kg, for a few biomass qualities
You will notice that the span covered extends across approximately a factor of 2 from about 14-15 up to just below 30. The ash content is even more variable, extending from well below 1 % by weight in stem wood up to about 20 % in rice husk and some other agricultural by-products and even more in some industrial wastes such as formwork timber. The handling during harvesting/collection and during transport may, as already mentioned, increase the total ash content by several % and the indicative values given here are the intrinsic values.
The effective (lower) heating value of a substance is
qNET = qNET,DAF * (1-fASH) * (1-fW) – 2.443 * fW MJ/kg.
Expanding this expression yields
qNET = qNET,DAF * (1 – fASH – fW – fW * fASH ) – 2.443 * fW MJ/kg
and this equation reveals that the most important factor to determine the heating value is the moisture content.
The moisture content in biomass is of two different kinds. A fraction of the water is bound to the cell walls by hydrogen bonds while the water content exceeding this amount is present as free water contained in the cell cavities.
The amount of water bound to the cell walls, typically about 15-25 % by weight on a wet basis, is called the amount of water at fibre saturation and depends on the type of biomass. Since this water is chemically bound it requires some extra energy to be evaporated and to obtain a completely dry biomass sample the material requires drying at elevated temperature, European federal standard prescribes 105 °C, for a long time, 24 hours.
The free water, the water exceeding the water content at fibre saturation, requires less energy for its vaporisation.
Drying can be accomplished mainly in three different ways:
• Open-air drying is the cheapest and simplest method. The biomass needs to be exposed to sun and air while, at the same time, it is protected from rain and from wet soil. Hence, the biomass must be piled in porous piles, oriented to be exposed to wind and sun but covered to be rain protected. This can be applied for such biomass fractions that lend themselves to build porous piles, such as tree branches or treetops, i.e. felling and forestry residues. For straw and some other types of agricultural side-products such as stalks, tops and haulm, the most common is to leave the material evenly distributed in the field for a few days. In favourable conditions and provided sufficient time, open-air drying may bring the biomass down to equilibrium with the surrounding atmosphere. The equilibrium may even be at lower moisture content than the fibre saturation point.
• Mechanical de-watering can basically be accomplished either by centrifuging, a common method with sludge, or by mechanical pressing, often applied with wet bark and/or with some agricultural residues. What can be achieved via mechanical de-watering is typically limited by the mechanical energy input but for practical applications moisture contents after de-watering are usually still higher than 50 %.
• Forced drying is the most expensive method requiring a forced draft of air to be supplied through the material. The material must be permeable to the air stream to avoid too high requirements of pressure with the fan and the air supplied can be at ambient temperature or at an elevated temperature. By forced drying, the final moisture content can be pre-determined and the drying process can be controlled.
If possible, and depending on the type of biomass, the supply chain should be planned to include open-air drying.
03-00-02d: Unit operations – Comminution
Almost regardless of the process – be it combustion, gasification, digestion, fermentation or any other process – the solid biomass will need to be fragmented to enhance process performance. Fragmentation – for example of tree branches – will also radically increase the bulk density when the material is loaded on to a carrier and will hence improve transport economy as outlined above.
Different types of materials, as well as different demands on the final particle size, will require different types of equipment but without going into too much detail one may distinguish four major types of machinery commonly used.
• Chippers. There are several different types of chippers but common to them is that they typically use sharp tools, “knives”, to cut the material into smaller pieces. The output from chippers is typically in the size range 5 – 50 mm. With some designs, the material is even coarser and the chippers producing such coarse fractions, chunks of material, are sometimes referred to as chunkers. Chippers are commonly used with relatively hard and coarse materials such as woody biomass. A well-designed chipping process may produce particles that are about equilateral so that the ratio between the tallest and the shortest measure of length, width and height is less than about 5. Equilateral particles will improve the performance with subsequent feeding of the particles into any type of reactors. The energy requirement for chipping is to a great extent depending on the shear strength of the material chipped.
• Shredders. While the fragmentation principle of a chipper is usually that of cutting the principle of a shredder is rather to tear the material into longer or shorter strands or strips. Since the principle of a shredder is hence completely different from that of a chipper it is clear that the shredder will usually produce a material where the length of the particles is commonly much greater than any of the two cross-sectional measures. So particles will become needle-like. The energy requirement for shredding is strongly depending on the tensile strength of the material.
• Mills. The most common type of mill in these applications is the hammer mill. In the hammer mill, fragmentation is obtained by rapid impact on the particles and the specific energy requirement will be strongly depending on the hardness of the material. The size of the product is determined by a sieve at the mill output and can be anything in the size range from 0.01 – 5 mm.
• Crushers. Crushing is an operation in which particles are fragmented through a relatively slow compression process unlike the rapid impact occurring in hammer mills. Fragile and brittle materials, such as coal or coke, are commonly milled in ball mills or rod mills. In these types of mills the actual process is crushing. For some assortments of biomass where solid mineral contaminants may be present, demolition wood, stumps, formwork timber, crushing between blunt tools such as in a jaw breaker may be the best alternative. In case of co-firing biomass with coal and if the biofuel is delivered as pellets or briquettes the biomass may be milled together with coal in ball- or rod mills. The prerequisite for crushing to work is that the material is brittle.
It is obvious that the total energy requirement for comminution is not only depending on the material but also on the size reduction and on the construction.
The intrinsic mechanical behaviour of biomass strongly depends on moisture content and also on the origin of the biomass and it is very difficult to suggest what is a reasonable energy consumption for a comminution process in any specific case. However; if the energy consumption for fragmenting exceeds about 150 kJ/kg of material there may be reason to re-consider the process used.
After fragmentation – and depending on the technology used – a sizing of the material may be a natural step. The need of sizing depends strongly on the process step succeeding the fragmentation. In most application, a common vibrating sieve will be sufficient but it must be appreciated that the different comminution methods will produce particles of very variable shapes, so the mesh is not necessarily a relevant measure. In some applications, especially in combination with pulverized fuel firing, may an air classifier be a better choice. For some more information about the difficulties associated with biomass sizing, please refer to text section 03-00-02h about conveying.
03-00-02e: Unit operations – Storage
Storing is an unavoidable step in any solid biomass supply chain. The problems associated with storing do mainly come back to the fundamental fact that biomass is degradable. Degradation processes start the very moment the biomass is harvested and cannot – in general terms – be avoided. However, there are some factors that are advantageous to degradation and such factors should, as far as possible, be eliminated or at least avoided.
Generally speaking one may distinguish two main routes for biodegradation:
• Aerobic degradation. Aerobic degradation occurs when the biomass is readily accessible for molecular oxygen, i.e. air, and ultimately results in the biomass being completely converted to carbon dioxide and water vapour. In popular terms this process may also be called composting.
• Anaerobic degradation. Anaerobic degradation may occur if the biomass store is depleted from oxygen because it is so compact as to prevent ventilation or if it is stored in a closed compartment.
For anaerobic degradation, it must be noticed that this can also be used as a method for biomass upgrading, i.e. for the transformation of a sludge into (bio-)methane or of a sugar-containing residue into (bio-)ethanol. Such upgrading processes are treated in chapters 01-03, 02-03, 03-03, 04-03 and 05-03 respectively.
For both processes to start, it is necessary that the simplest sugar compounds in the cell liquid are readily accessible for micro-organisms. This occurs once wet biomass is fragmented and hence it is true to say that the first condition to favour biodegradation during storage is to store fragmented and wet biomass.
Typically, both processes will start by mould fungi establishing in the material. Mould is tolerant to wide limits of humidity and oxygen and since mould spores are omnipresent, this establishment will occur within days after the biomass pile has been built. The types of mould may be different depending on the material stored and on the climatic zone, but they will be there.
Mould fungi can generally not digest lignin but mainly feed on starch, sugars, cellulose and hemi-cellulose. Some species, though, do produce an enzyme that degrades lignin into simple sugars. However, the establishment of mould has several consequences in the storage pile:
1) Heat is released. The heat release will induce a natural draught through the material. If the pile is porous the draught may be large enough to maintain a relatively high oxygen concentration in the central parts of the pile and also to transport away the excess heat so that the temperature is maintained at a low level. In case the pile is more compact, the inner parts of the pile may be depleted from oxygen and the temperature may rise.
2) In case of a temperature increase, water will vaporize and the central parts of the material will successively become drier. At the same time, it must be remembered that the fungi themselves produce new water vapour by their digestion, so there is not only a drying but also a restoration of moisture. The relatively high vapour pressure in the central part of the pile will in turn lead to a re-distribution of moisture so that the outer and cooler parts of the pile experience successive increase in water content.
3) The rapidly assimilable fraction of the organic material is consumed.
The above processes will cause a successive change of the living conditions in the material and new species of fungi and bacteria will successively establish. It is also clear from what was said in the first point above that the conditions may change from aerobic to anaerobic, depending on the porosity of the material. At the same time, there will be an increase of the water content in the outer parts of the pile where the temperature is strongly influenced by the surrounding.
The increasing water content in parts of the pile may – in combination with the temperature increase – provide good living conditions for rot fungi and -bacteria, different species depending on the material stored. Rotting will typically not only affect the biomass content of cellulose and hemicellulose but will also consume lignin and other more complex substances.
To minimize degradation during storage it is important that the material is stored in such a form that promotes ventilation, i.e. that the increase of temperature mentioned in point 1 above is minimized. For some types of biomass, such as tree branches, this is easy to accomplish simply by storing the material in its original form. For other types of biomass – such as maize leaves – a porous pile cannot be established unless some type of scaffolding is erected.
Some types of biomass, such as household waste, are best stored in air-tight plastic bags resembling those used for silage. This reduces the possibility for aerobic degradation and also minimizes the risks for malodour spreading and also keeps rodents under control.
In case high porosity cannot be obtained, the piles will instead have to be low enough that natural draught can be established through them. For agricultural residues this height is only a few meters, 1-3 m at the most, and the storage will require a large land area. For wet wood chips, mixed fractions including bark, may the height be up to 6-7 m while for pure stem-wood chips the height may be up to 15 m. Shredded wet bark, which will form compact piles, may only be stacked up to about 3-4 m, just like most agro-residues.
To minimize losses during storage it is important to keep different fractions of biomass separate as long as possible during the supply chain. Different fractions will, typically, be attractive to different types of micro-organisms and will offer different types of advantages. So may one type of biomass offer easily digestible sugars while another assortment offers higher moisture content. Mixing the two fractions will then create an environment where both easily assimilable sugars and water are both present in large amounts and these enhanced living conditions will attract macro-organisms and increase the biological deterioration and the losses.
While a proper storage may exhibit losses of combustible material – i.e. of the total energy content – less than 1 % per month an improper storage may lead to total losses in the order of magnitude 10 % per month or even more.
03-00-02f: Unit operations – Homogenization
Regardless of how well the supply chain may be designed there will always be a need of short-time storage near to the energy plant. As pointed out in the previous text section, this short-time storage should best be designed so as to keep different assortments separate. But the process – be it combustion, gasification, biochemical conversion or whatever – is generally speaking best operated on a reasonably constant feedstock quality. Hence, the material needs be homogenized prior to feeding.
Homogenization may aim at two major aspects:
• Homogeneity with respect to particle size and shape
• Homogeneity with respect to average composition, including moisture content
The former is best achieved by mixing the raw materials just prior to fragmentation and, if the demands on homogeneity are extremely high, by a subsequent compaction into pellets, see the next text section.
For the latter it must be remembered that as soon as biomass is left by itself, the biological deterioration commences and that this deterioration (see text section 03-02-05 about storage) will lead to an internal re-distribution of moisture in the pile and to a non-homogenous material. Hence, mixing must be postponed as long as possible and should preferably be done by the front-loader driver just prior to feeding the material to the day-hopper and not earlier. For the stability of the subsequent process, it is therefore essential that the driver of the front-loader is properly instructed. He or she will have a very important role to play as it comes to the stability and to the performance of the combustion/gasification/… process.
03-00-02g: Unit operations – Compaction
As mentioned in 03-02-02, the bulk density is one determining factor for the energy density and for transport economy. The bulk density is determined by two factors, namely the material density itself and the degree of packing obtained when the material is loaded.
In some applications, when the hauling distance is long, pelletizing or briquetting may be worthwhile prior to transport. For example the bulk densities of stubbles or cutter shavings are typically less than 100 kg/m3. If the material is pelletized, the bulk density is increased to about 6-800 kg/m3. With briquettes the corresponding values are about 3-500 kg/m3.
In pelletizing, the material must first be milled to a suitable particle size – different depending on the material – and then isostacially compressed to form durable particles of a uniform shape. To make pellets, the isostatic pressure must be high enough to completely break the cell structure, for lingo-cellulosic biomass typically about 700 bar. For briquettes, the demands on mechanical durability are lower and the necessary pressure is only about 150-200 bar.
Another technique is baling. Baling is typically applied in close connection with the harvesting/collection process and does not involve milling.
03-00-02h: Unit operations – Conveying
A stable feed is essential for any process, be it combustion, gasification, biochemical treatment or anything else.
The typical process layout with a biomass energy system will be that there is an intermediate outdoor store on-site, that the material is mixed and fed to a final fragmenter, conveyed to a day hopper and from that is finally fed to the process. So the process chain will contain three different conveying steps:
• Conveying the un-fragmented material
• Conveying fragmented material
• Emptying the hopper
Depending on the biomass may the un-fragmented material be more or less non-uniform in shape and size. Characteristic of biomass is that it is fibrous and that that it has a low density. The fibrous or ropy structure will often result in a tenacious material with knots and in some cases, like in the presence of bark strands may the material be sticky enough to twist around the feeding screws and actually stop the feeding completely. Similarly may an ever so well controlled collection of a clean household waste fraction contain kitchen pans or pantyhose to stop the feeders. With biomass originally collected from the ground – residues from felling sites, agricultural residues etc. – will stones be present more or less frequently. With demolition wood one will have to expect chunks of concrete, nails, screw, hinges and things like that.
In case screws are used and to guarantee a smooth operation of the primary feed it is therefore essential first that the drive motor for the screws is dimensioned with sufficient power and second that the diameter and the pitch of the primary screws are large enough to handle anything that may be present in the fuel.
In connection to the fragmentation it is common also to have a separation of foreign material such as stones from the biomass. Sizing may also be applied in connection to fragmenting and is then organized so that oversize material is returned to the fragmenter.
After fragmentation the size range of the material is narrowed as compared to the raw material. With the low density of biomass this means that the particles are lighter and with the fibrous structure it also means that the particles will often have a ragged surface. These things combine to make friction between the particles a dominating property causing comminuted, raw biomass to have very steep repose angles, in some cases even negative. This, in turn, means that mass flow control of the material becomes very difficult since for example a conveyor belt will not be filled to the same level all the time nor will the volume transported through a screw be constant.
Also will the lightness of the material set a limit to the inclination angle for flat conveyor belts at about 30–40° to horizontal. With centreless screws the inclination may be steeper, about 60° to horizontal, except for pellets where again the limit is about 30–40°.
The design of biomass hoppers must take the repose angles into due account. The low density of the individual particle in combination with the ragged surface makes raw biomass have a high tendency for bridging. To avoid this, hoppers should be designed to expand downwards, so they should be designed with a negative slope of the walls. For pellet hoppers exceeding about 1 m in cross section, this is not necessary but the hopper may be designed “the normal way” as long as the bottom angle is steeper than 45° to horizontal.
03-00-02i: Unit operations – Quality control
On arrival at the receiving plant, the biomass must undergo quality control. The quality control aims at determining the total amount of biomass delivered, the energy content of the biomass delivered and, perhaps, the ash content and/or –properties.
In many cases the final transport to the energy plant will be road transport by truck and the solid material will be present in containers. There are then two different ways to determine the total amount delivered namely volume measurement or weight measurement.
The method for volume measurement is basically to estimate the degree of fullness in the containers and to use the known container volume in combination with this factor to determine the volume delivered. Since the bulk density cannot be accurately determined, this method will involve two uncertainties, namely the uncertainty in volume and the uncertainty in bulk density and the total amount received will then suffer from a total uncertainty equal to the sum of these two. Experience values from Swedish plants reveal that the total uncertainty with this method is in the order of magnitude + 10 % or even more. The applicable European federal standard is EN 15103.
Weight measurement, weighing the loaded truck on arrival and the empty truck when leaving, provided of course that the scale is properly calibrated, reduces the total uncertainty to less than + 1 %.
For moisture content as well as for ash properties the degree of uncertainty in the final value all comes back to the sampling. Picking only few samples from the top of the container is not enough to guarantee that the total sample is representative for the load. Again, there are European standards available: EN 14778, 14779 and 14780.
03-00-03: Risks and hazards
By now, it is clear that the supply of biomass is a complex process involving a number of different steps. Each of these steps will contain its own set of critical moments as will now be outlined.
For economic reasons, most biomass transports will involve comminuted biomass, i.e. particles in the size range 5 – 250 mm, loaded in open containers. Since the particles are thus small and have a low density they will easily be blown off the truck. To avoid particles blowing off and hitting following cars, the containers must be covered with nets or, better, with tarpaulins. Tarpaulins will also reduce the amounts of road dust depositing in the fuel and will hence reduce the ash content in the final delivery.
Except from the obvious risks involved in chipping and milling operations, as well as those involved in loading and unloading, the major risks with biomass handling are connected with the storing.
There are mainly two risks involved in the storing process:
• Auto-ignition
• Allergenic reactions
The micro-organisms, fungi as well as bacteria, responsible for the degradation of biomass during storage may be classified into three major groups: cryophilic, mesophilic and thermophilic. While cryophilic organisms thrive at low temperatures (about -15 – 15 °C) mesophilic organisms do prefer higher temperatures (about 5 – 50 °C) and thermophilic organisms thrive at high temperatures (ranging up to above 100 oC). Thus biochemical processes may increase the internal temperature in a storage pile all the way up to temperatures about 100 °C. If conditions are favourable, correct oxygen content, correct flow rate of air, and if an oxidation catalyst is present – iron, chromium, nickel, all common elements in high alloy steels – chemical oxidation may occur already at these low temperatures. The catalyst may not even be necessary if the biomass contains sufficient amounts of unsaturated fatty acids. For example a porous structure – such as a twist of cotton waste – may self-ignite if it is impregnated by linseed oil. However, the presence of a catalyst, such as any piece of alloy steel, will radically increase the risk of auto-ignition.
Internal combustion by auto-ignition in a pile of wet organic material is not necessarily visible on the outside. The internal of the pile may well have temperatures about 2-300 oC and be carbonized while the outer 50-100 cm looks quite normal. Opening up the pile with a front-loader and hence providing oxygen access to the internal pats will immediately lead to flaming combustion. But it is also important to remember that a normal looking pile may well be – effectively – a charring stack and that it is strictly prohibited to ever step or drive on top of storage piles.
The successive development in the stack, as outlined in section 03-02-05, includes a successive migration of mould fungi from the most central parts of the pile and approaching the surface as the living conditions alter. As the conditions in one part of the stack become too hostile and the fungi migrate, what they do is to leave spores after them. Hence the pile of material may well contain strata that are heavily contaminated by mould spores. Opening up the stack with a front loader will release these spores into the working atmosphere and the machine driver will exposed to extremely high concentrations of potentially pathogenic and allergenic spores.
For some types of biomasses there is also a risk of pathogens forming during storage. This is most pronounced with biomasses intended for biogas production or for ethanol fermentation, i.e. with such biomasses that are specially selected to be easily biodegradable. Special care must hence be taken at such plants to avoid infection and poisoning.
03-00-03a: Risks with closed compartments
Sometimes, for example during inter-continental shipping, biomass such as pellets, briquettes or chips may be stored in closed compartments. Storing in silos and in hoppers are other examples commonly used.
Since biomass is always a living material and never becomes inert, neither from a chemical nor from a biological standpoint, the atmosphere in the closed compartment will become depleted from oxygen and may contain not only explosive gas components but also carbon monoxide. Hence the atmosphere inside the compartment may be suffocating and/or explosive and/or poisonous.
Before entering such a compartment – for example the hold on a cargo ship to assist unloading – the compartment must be thoroughly ventilated. It is important to understand that this holds true not only for fresh biomass but also for dry wood pellets, briquettes and all other qualities.
03-00-04: Planning aspects
Planning for a reliable supply of biomass becomes very different depending on the resource, please refer back also to text section 02-00-03.
Looking first at the availability side of things one may split the main resources into three groups:
Annual crops, i.e. herbaceous or agricultural resources, are annual and the actual yield a specific year is strongly weather dependent. Since the mean annual yield (MAI) is per definition a value that is in the middle of the variation span, planning for the MAI will yield a deficit of fuel every second year (on average) while there will be a surplus every second year. It can also be assumed that the weather conditions are regional, so that a deficit in one region a certain year will correspond to a deficit also in the neighbouring regions the same year. Similarly, surplus in one region will co-variate with a surplus in the neighbouring regions. The consequence of this is that if the mean annual yield is used as a basis for planning, the plan will in itself be one to promote strong annual price fluctuations.
To be on the safe side, so that the most common situation is one where fuel is abundant and price is kept low and stable, the over-all plan should not be allowed to make long-term use of more than the mean annual yield subtracted with the standard deviation as calculate from at least ten years statistics.
Societal, industrial and livestock resources, such as sorted waste fractions, fibre sludge, manure or similar residual products are not strongly weather dependent but will exhibit a variation due to the state of the market and to the consumption patterns. The annual variations in these resources will typically be slower and less extreme and they may also, to some extent, be predictable.
To be on the safe side, so that the most common situation is one where fuel is abundant and price is kept low and stable, the over-all plan should not be allowed to make full long-term use of the mean annual yield but there should be a margin.
Perennial crops, such as wood and forestry residues, will reflect the industrial structure of the region but will be depending on the actual state of the sectorial market, too. Hence, there will again be variations to the supply side of the market and the planner needs take this into due account.
To be on the safe side, so that the most common situation is one where fuel is abundant and price is kept low and stable, the over-all plan should not be allowed to make full long-term use of the mean annual yield but there should be a margin.
But not only will the supply side need to be taken into account.
The user-end, the process chosen, will also have an influence on the planning:
Processes involving biochemical treatment (fermentation or anaerobic digestion) requires that the feedstock is easily accessible for biological treatment – i.e. this biomass is per definition not suitable for long-term storage. Thus, variations in availability cannot effectively be met by storing unless precaution is taken to make the feedstock more-or-less biologically passive during the storage time.
Processes flexible with respect to moisture – mainly direct combustion – will to some extent suffer from the same problem. Wet biomass stored in a pile will provide excellent living conditions for a large number of micro-organisms and, as pointed out above (section 03-00-02e), deterioration starts the moment the pile is erected.
Processes demanding a dry fuel – this will include several thermochemical conversion processes – will be less susceptible to storage since dry material will deteriorate slower. However, this will demand that the material is stored rain-protected ant that the material is not hygroscopic.
The categories can be illustrated and roughly classified in the form of a matrix:
PUTRESCIBLES | SOLID MATERIAL | ||||
WET | DRY | ||||
Manure, Sludge etceteras | Herbaceous material | Woody and waste fract. |
Herbaceous material | Woody and waste fract. |
|
Fermentation Anaer. digestion |
Amount and price sensitive to long-term market | Amount and price both sensitive to weather | |||
Resource not suitable for storage | Resource not well suited for storage | ||||
Direct combustion |
Amount and price both sensitive to weather | Amount and price both sensitive to market | Amount and price both sensitive to weather | Amount and price both sensitive to market | |
Resource not well suited for storage | Wood can be stored for some time | Resource can be stored | Resource can be stored | ||
Thermochemical conversion |
Amount and price both sensitive to weather | Amount and price both sensitive to market | Amount and price both sensitive to weather | Amount and price both sensitive to market | |
Resource not well suited for storage | Wood can be stored for some time | Resource can be stored | Resource can be stored |
Blank | Material not well suited for this process |
Material can be used in this process | |
Material very well suited for this process | |
Table 03-00 2: Very rough classification of biomasses and processes |
Biochemical treatment
The table is by no means definite but serves to illustrate that fermentation or digestion based on the regional livestock population and/or on the sludge produced at the city wastewater treatment plant and/or on the sludge produced by regional industry may be trusted to have a reasonably constant supply of feed but that the plant will also have to be dimensioned to handle the maximum amounts expected. If a substantial part of the feed originates from livestock, it will be sensitive to the market demand and to the livestock population.
Fermentation and digestion processes will also be able to accommodate a certain amount of wet crops or crop residues as additives to the substrate depending on quality. Since this fraction will be subject to stronger price fluctuations it may be used as an economic balance – as long as the process performance is not at stake. However, wet crops tend to be susceptible for deterioration and may cause problems during storing. Please refer to chapter 04-03 for further detail.
Provided the feed to a biochemical conversion plant is clean, i.e. free not only from heavy metals but also free from pathogenic bacteria, organic toxins and other harmful substances, the sludge may be used as a fertilizer. The quality control, of the sludge as well as of the feed, will be subject to federal and national law.
Direct combustion
Direct combustion – in which 100 % of the fuel energy is released in one operation – will usually lead to high temperatures in the combustion chamber. The equipment will be designed to handle different moisture contents but for the combustion process to go to completion, local temperatures will have to exceed about 1100 °C. The combustion chamber can be designed to handle fuels with more than 50 % moisture, so for this reason also wet crops and crop residues would be an acceptable fuel – but many crops exhibit low ash melting temperatures, as low as 700 °C, or high contents of chlorine, more than 0.5 % by weight has been reported for several types of straw, and sulphur and may thus cause process problems as well as emissions in combustion applications. Drying the material reduces the storage problem but not the problem with corrosive gases and ash melting. The ash properties and possible content of chlorine, sulphur and other trace elements are the reasons for the orange colour in the table.
Woody biomass, in most cases, will exhibit higher ash melting temperatures – often more than 1100 °C – and with a properly designed combustion chamber ash melting can be kept under control. Woody (ligno-cellulosic with a high lignin content) biomass also deteriorates slower than the softer and more porous herbaceous biomass and is hence less susceptible to storage. If dried to moisture contents below fibre saturation, see text section 03-00-02c, deterioration is slowed down significantly and – if kept dry – the material can be stored for a long time.
If a combustion plant is fed with too dry a fuel temperature may rise too high and damage the plant but this can be compensated by fuel conditioning using a water spray prior to the feed. Hence, a lack of fuel with optimal moisture content can be compensated with addition of a drier fuel followed by fuel conditioning.
A more severe situation is the case that the plant is designed for dry fuel or for pellets or briquettes. In these cases, a fuel deficit cannot easily be compensated by addition of another fuel quality but the correct quality will have to be acquired from elsewhere.
Direct combustion of societal or industrial waste fractions will be subject to federal and national law.
Thermochemical conversion
In thermochemical conversion only part of the energy input is released, sufficient to reach the process temperature necessary, and the remaining feed energy is retained in the product fuel. If the feed is too wet too much fuel will be consumed to reach the process temperature and the process will become un-economic. Thus fuels suited for thermochemical conversion are only those that are reasonably dry, typically less than about 30 % moisture content.
To retain the largest possible fraction of the input energy in the product fuel, process temperatures are kept as low as possible during any thermochemical conversion and the low ash melting temperatures with crops and cop residues become less of a problem than in direct combustion.
The constraint on moisture content restricts the use of wet biomass in thermochemical conversion to amount not violating the moisture condition. The same holds true for clean waste fractions.
However – depending on the process actually used – trace elements such as sulphur, chlorine and alkali metals may pose severe problems in subsequent gas processing. This is most pronounced in case of gas synthesis involving catalysts but may also be prohibitive in case the subsequent combustion process is combined-cycle power production.